Steel structures play a vital role in modern construction, providing strength, durability, and versatility in various applications, including buildings, bridges, and industrial facilities. Among the critical components of steel structures are compression members, which are structural elements designed to resist compressive forces. These members are essential in maintaining the overall stability and structural integrity of the system.
What are Compression Members?
Compression members are structural elements that primarily resist compressive forces acting along their longitudinal axes. These forces can arise from various sources, such as gravitational loads, wind loads, or seismic forces. Compression members are typically used as vertical supporting components, transferring loads from above to the foundation or other load-bearing elements below.
Importance of Compression Members in Steel Structures
Compression members are indispensable components in steel structures for several reasons:
- Load Transfer: They facilitate the transfer of gravitational and lateral loads from upper levels to the foundation, ensuring the stability and structural integrity of the entire system.
- Lateral Resistance: Certain types of compression members, such as braces and struts, play a crucial role in providing lateral resistance against wind and seismic forces, enhancing the overall structural resilience.
- Versatility: Compression members can be designed and fabricated in various shapes, sizes, and configurations, allowing for architectural flexibility and efficient use of materials.
- Construction Efficiency: The use of compression members in steel structures enables rapid construction processes, reducing overall project timelines and associated costs.
Types of Compression Members
Compression members in steel structures can be categorized into several types based on their geometrical characteristics, load-carrying behavior, and applications.
Short Columns
Characteristics:
Short columns, also known as stocky columns, are compression members with a relatively low slenderness ratio (the ratio of the effective length to the least radius of gyration). They are typically characterized by their ability to resist compressive forces primarily through direct compression, with minimal susceptibility to buckling effects.
Applications:
Short columns are commonly used in applications where the primary load is compressive, such as:
- Lower levels of multi-story buildings
- Support columns for machinery and equipment
- Structural members in industrial facilities
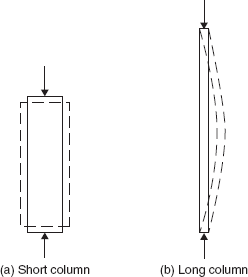
Long Columns
Characteristics:
Long columns, or slender columns, have a higher slenderness ratio compared to short columns. Due to their increased slenderness, long columns are more susceptible to lateral deformations and buckling under compressive loads. Their design considerations must account for the potential for buckling failure modes.
Applications:
Long columns are often employed in structures where significant vertical loads need to be transferred over considerable heights, such as:
- Upper levels of high-rise buildings
- Transmission line towers
- Offshore platforms
Struts and Braces
Struts and braces are compression members that are primarily responsible for providing lateral stability and resistance against horizontal forces.
- Struts: Inclined or diagonal compression members that transfer lateral forces to other structural elements or foundations.
- Braces: Compression members that are part of a bracing system, typically arranged in diagonal or chevron patterns, to resist lateral loads.
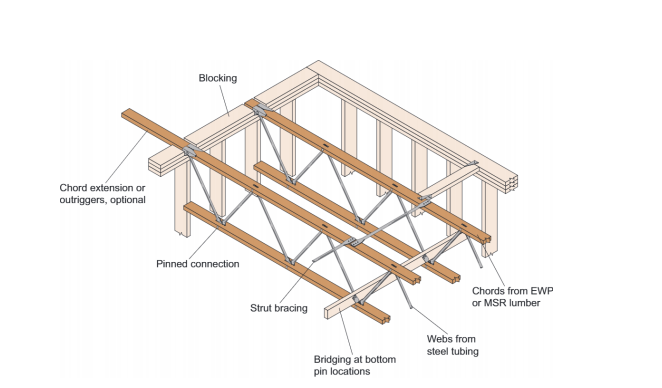
Applications:
Struts and braces are widely used in various steel structures, including:
- Braced frames in high-rise buildings
- Truss systems in bridges and industrial structures
- Lateral bracing systems in storage racks and towers
Built-up Columns
Types:
Built-up columns are compression members that are fabricated by combining multiple structural elements, such as plates, channels, or angles. They can be classified into two main types:
- Laced Columns: Composed of two or more structural members connected by lacing bars or tie plates.
- Battened Columns: Constructed by connecting two or more structural members using batten plates or intermittent spacers.
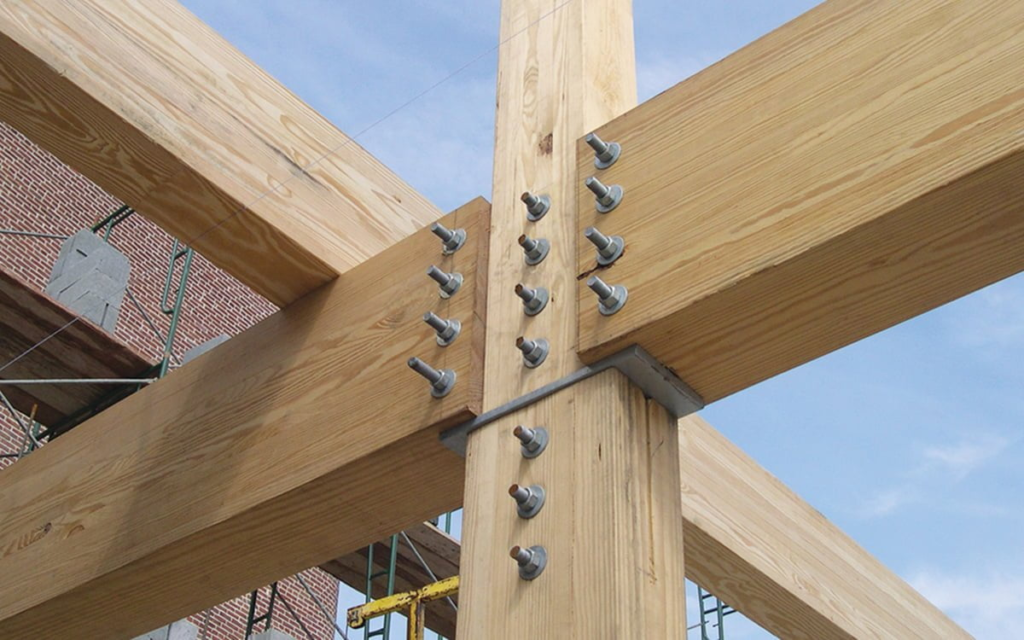
Advantages and Uses:
Built-up columns offer several advantages over solid columns, including:
- Efficient use of materials by optimizing the cross-sectional area
- Ability to achieve larger cross-sections for increased load-carrying capacity
- Improved lateral stability and resistance to buckling
- Commonly used in industrial structures, bridges, and high-rise buildings where heavy loads need to be supported.
Materials Used for Compression Members
The selection of materials for compression members in steel structures is crucial to ensure structural integrity and performance. The most commonly used materials are structural steel grades, which are specified by various standards and codes.
Structural Steel Types (e.g., ASTM A36, A992)
The American Society for Testing and Materials (ASTM) provides specifications for different types of structural steel, including:
- ASTM A36: A widely used carbon steel grade with a minimum yield strength of 36 ksi (248 MPa). It is suitable for various compression members and general structural applications.
- ASTM A992: A high-strength, low-alloy steel grade with a minimum yield strength of 50 ksi (345 MPa). It is commonly used in compression members for high-rise buildings and other structures subjected to heavy loads.
Other structural steel grades include ASTM A572, A913, and A500, which are specified based on their chemical composition, mechanical properties, and intended applications.
Material Properties Relevant to Compression Members
The key material properties that influence the design and performance of compression members include:
- Yield Strength: The stress level at which the material begins to deform plastically, affecting the load-carrying capacity and buckling resistance.
- Ultimate Strength: The maximum stress the material can withstand before failure, determining the maximum allowable load.
- Modulus of Elasticity: A measure of the material’s stiffness, influencing the buckling behavior and deflection characteristics.
- Ductility: The ability of the material to deform plastically without sudden failure, ensuring a more gradual and predictable behavior under excessive loads.
- Weldability: The suitability of the material for welding processes, which is essential for fabricating built-up compression members.
The selection of materials for compression members involves considering factors such as strength requirements, cost-effectiveness, and compatibility with other structural components.
Design Principles for Compression Members
The design of compression members in steel structures is governed by several fundamental principles to ensure structural safety, serviceability, and economy. These principles are based on theoretical concepts, empirical data, and industry standards.
Load Carrying Capacity
Axial Load:
The primary function of compression members is to resist axial compressive forces. The design must ensure that the applied axial load does not exceed the member’s load-carrying capacity, which is determined by factors such as cross-sectional area, material properties, and slenderness ratio.
Buckling Load:
In addition to axial loading, compression members are susceptible to buckling, a phenomenon where the member loses stability and undergoes lateral deformation under compressive loads. The design must account for the critical buckling load, which is influenced by factors such as the member’s effective length, slenderness ratio, and end conditions.
Effective Length
The effective length of a compression member is the equivalent length of an idealized column with the same flexural stiffness and end conditions as the actual member. It accounts for the boundary conditions and the stiffness of adjacent structural elements, which can affect the buckling behavior.
Calculation Methods:
Various methods exist for calculating the effective length of compression members, including:
- Theoretical Methods: Based on analytical solutions for idealized end conditions (e.g., fixed-fixed, fixed-pinned, pinned-pinned).
- Empirical Methods: Derived from experimental data and observations of actual member behaviors.
- Alignment Charts: Graphical representations of effective length factors based on the member’s end conditions and stiffness ratios.
- Numerical Methods: Finite element analysis or other computational techniques to model the member’s behavior and determine the effective length.
The accurate determination of the effective length is crucial for predicting the buckling load and ensuring the member’s stability under compressive loads.
Slenderness Ratio
Importance in Design:
The slenderness ratio, defined as the ratio of the effective length to the least radius of gyration, is a fundamental parameter in the design of compression members. It quantifies the member’s susceptibility to buckling and governs the applicable design equations and failure modes.
Impact on Strength:
As the slenderness ratio increases, the compressive strength of the member decreases due to the increased likelihood of buckling failure. Design codes and specifications provide different strength equations and limitations based on the slenderness ratio, ensuring that members with higher slenderness ratios are designed with appropriate considerations for buckling effects.
By considering the effective length, slenderness ratio, and load-carrying capacity, engineers can design compression members that meet the required strength and stability criteria while optimizing material usage and construction costs.
Buckling of Compression Members
Buckling is a critical failure mode that compression members must be designed to resist. It occurs when a structural element, subjected to compressive forces, becomes laterally unstable and undergoes significant lateral deformation. Understanding the types of buckling, the factors that influence it, and the methods to prevent it is essential for ensuring the safety and reliability of steel structures.
Types of Buckling
Euler Buckling (Elastic Buckling):
Euler buckling, also known as elastic buckling, is a phenomenon that occurs when the compressive load on a slender member exceeds a critical value, causing it to deflect laterally without any yielding or permanent deformation. This type of buckling is characterized by a sudden and dramatic lateral displacement of the member, and it is typically observed in members with high slenderness ratios.
The Euler buckling load is a theoretical value derived from the principles of elastic stability, and it is often used as a reference point for design calculations. However, in practice, most compression members exhibit inelastic behavior before reaching the Euler buckling load.
Inelastic Buckling:
Inelastic buckling occurs when the compressive stresses in a member exceed the yield strength of the material, leading to plastic deformations before the onset of buckling. This type of buckling is more common in stockier members with lower slenderness ratios.
Inelastic buckling is characterized by a gradual lateral deflection accompanied by yielding of the material. Unlike Euler buckling, which is a sudden and dramatic event, inelastic buckling exhibits a more gradual loss of stability and load-carrying capacity.
Factors Affecting Buckling
Several factors influence the buckling behavior of compression members, including:
Slenderness Ratio:
The slenderness ratio, which is the ratio of the effective length to the least radius of gyration, is a critical parameter in determining the susceptibility of a member to buckling. As the slenderness ratio increases, the member becomes more prone to buckling at lower compressive loads.
Material Properties:
The material properties, such as yield strength, modulus of elasticity, and ductility, play a significant role in the buckling behavior of compression members. High-strength materials with limited ductility may be more susceptible to sudden and brittle buckling failures, while more ductile materials can exhibit gradual and stable buckling behavior.
End Conditions (Fixed, Pinned, Free):
The end conditions of a compression member, which can be fixed, pinned, or free, influence the effective length and the buckling behavior. Fixed-end conditions provide greater restraint and stability, while pinned or free-end conditions increase the likelihood of buckling at lower loads.
Preventing Buckling
To mitigate the risk of buckling and ensure the structural integrity of compression members, various preventive measures can be employed:
Bracing:
Lateral bracing systems, such as diagonal braces or perpendicular members, can be used to provide lateral support and restraint to compression members, reducing their effective length and increasing their buckling resistance.
Cross-Section Modifications:
Altering the cross-sectional shape or introducing stiffeners can enhance the buckling resistance of compression members. For example, built-up columns with batten plates or lacing bars can significantly improve their stability and load-carrying capacity.
By understanding the types of buckling, the factors that influence it, and the preventive measures available, engineers can design compression members that meet the required strength and stability criteria while optimizing material usage and construction costs.
Analysis and Design Codes
The design and analysis of compression members in steel structures are governed by various national and international standards and codes. These codes provide guidelines, design methodologies, and requirements to ensure the safety, reliability, and consistency of steel structures.
Relevant Standards and Codes
AISC (American Institute of Steel Construction):
The AISC Specification for Structural Steel Buildings is a widely recognized standard in the United States and other regions. It provides comprehensive design requirements, material specifications, and analysis methods for steel structures, including compression members. The AISC code covers aspects such as load combinations, design strength calculations, and stability considerations.
Eurocode 3:
Eurocode 3, also known as EN 1993, is a European standard for the design of steel structures. It is divided into several parts, with Part 1-1 covering general rules and rules for buildings, and Part 1-8 specifically addressing the design of compression members. Eurocode 3 provides detailed guidelines for buckling resistance, effective length determination, and cross-sectional classification.
IS 800 (Indian Standard):
In India, the design of compression members in steel structures is governed by the Indian Standard IS 800, which is based on the limit state design philosophy. This standard covers various aspects of steel construction, including material specifications, design principles, and load combinations for compression members.
Design Approaches
The design of compression members typically follows one of two primary approaches: Load and Resistance Factor Design (LRFD) or Allowable Stress Design (ASD).
Load and Resistance Factor Design (LRFD):
The LRFD approach is a semi-probabilistic method that accounts for uncertainties in both loads and material strengths. It involves multiplying the nominal loads by load factors and dividing the nominal resistance by a resistance factor. The design is considered satisfactory when the factored loads do not exceed the factored resistance. LRFD is the preferred method in modern codes and provides a more consistent level of safety across different structural elements.
Allowable Stress Design (ASD):
The ASD approach is based on the concept of permissible stresses, where the calculated stresses due to applied loads must not exceed the allowable stresses specified by the code. This method incorporates factors of safety to account for uncertainties in loads and material strengths. While still used in some older codes, ASD is generally being phased out in favor of the more modern LRFD approach.
These standards and codes provide comprehensive guidelines for the analysis and design of compression members, ensuring that steel structures meet the required safety, serviceability, and durability criteria. Engineers must stay up-to-date with the latest code revisions and adhere to the applicable standards for their specific projects and regions.
Common Problems and Solutions in Compression Members
Despite careful design and analysis, compression members in steel structures can be susceptible to various problems and failure modes. Understanding these common issues and their solutions is crucial for maintaining the structural integrity and safety of steel structures.
Local Buckling
Identification:
Local buckling occurs when the individual components or plates of a compression member, such as the flanges or web of a built-up column, experience instability and buckle locally. This type of buckling is typically observed in slender elements or members with thin-walled cross-sections.
Solutions:
To mitigate local buckling, the following solutions can be implemented:
- Cross-Section Stiffening: Introducing stiffeners, such as batten plates, lacing bars, or intermittent spacers, can increase the local stability of individual components and prevent premature local buckling.
- Cross-Section Modification: Altering the cross-sectional shape or dimensions can improve the local buckling resistance of compression members. For example, using thicker plates or closed cross-sections can enhance the local stability.
- Material Selection: Choosing materials with higher yield strengths or utilizing composite materials can increase the local buckling resistance of compression members.
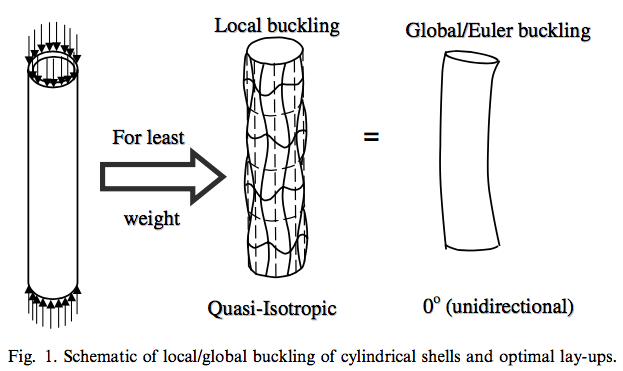
Global Buckling
Identification:
Global buckling, also known as overall buckling or flexural buckling, occurs when the entire compression member undergoes lateral deflection and instability. This type of buckling is primarily influenced by the member’s slenderness ratio and end conditions.
Solutions:
To address global buckling, the following measures can be taken:
- Bracing Systems: Implementing lateral bracing systems, such as diagonal braces or perpendicular members, can provide lateral support and reduce the effective length of the compression member, increasing its global buckling resistance.
- Increasing Cross-Sectional Dimensions: Increasing the cross-sectional dimensions or using built-up members can enhance the global buckling resistance by reducing the slenderness ratio and increasing the radius of gyration.
- End Condition Modifications: Improving the end conditions of the compression member, such as providing fixed or semi-rigid connections, can increase the critical buckling load and prevent global buckling.
Connection Failures
Common Issues:
Inadequate connections between compression members and other structural components can lead to premature failures and compromise the overall structural integrity. Common issues include:
- Insufficient Bearing Capacity: Inadequate bearing area or bearing stresses at the connections can cause local crushing or deformations, leading to connection failures.
- Bolt/Weld Failures: Improper sizing or placement of bolts or welds can result in inadequate load transfer and potential failures at the connections.
- Geometric Imperfections: Misalignments or eccentricities in the connections can introduce unintended bending moments or eccentric loading, increasing the risk of connection failures.
- Corrosion and Deterioration: Over time, connections can be susceptible to corrosion, material degradation, or fatigue, weakening their load-carrying capacity.
Strengthening Techniques:
To address connection failures and enhance the performance of compression members, various strengthening techniques can be employed:
- Reinforcement Plates: Adding reinforcement plates or stiffeners at the connections can increase the bearing area and distribute the loads more effectively, reducing localized stresses.
- Upgrading Fasteners: Replacing existing bolts or welds with higher-strength fasteners or increasing the number of fasteners can improve the load transfer capacity of the connections.
- Introducing Braces or Ties: Installing additional bracing members or tie elements can help redistribute the loads and provide additional support for the connections.
- Protective Coatings: Applying corrosion-resistant coatings or enclosures can prevent deterioration and extend the service life of the connections.
- Connection Replacement: In severe cases, complete replacement of the connections may be necessary, using improved designs or materials to meet the required performance criteria.
By addressing common connection issues and implementing appropriate strengthening techniques, engineers can ensure the structural integrity and reliability of compression members in steel structures.
Case Studies and Real-World Applications
Compression members play a crucial role in various real-world applications, from high-rise buildings and bridges to industrial structures. Examining case studies and real-world examples can provide valuable insights into the design considerations, challenges, and solutions employed in these applications.
High-Rise Buildings
Examples of Compression Members in Skyscrapers:
In high-rise buildings, compression members are extensively used to transfer the loads from the upper levels to the foundation. Some notable examples include:
- Burj Khalifa, Dubai: The world’s tallest building at 828 meters, features a reinforced concrete core supported by a system of compression members, including built-up columns and diagonal braces.
- Shanghai Tower, China: Standing at 632 meters, this skyscraper incorporates a composite construction with steel compression members, such as mega-columns and outrigger trusses, to resist lateral loads.
- One World Trade Center, New York: The main structure of this 541-meter tower includes a central reinforced concrete core surrounded by steel compression members, including built-up box columns and braces.
Bridges
Role of Compression Members in Bridge Design:
Compression members play a vital role in the design and construction of various types of bridges, including:
- Truss Bridges: In these bridges, the top and bottom chords act as compression members, transferring loads to the supports and resisting bending moments.
- Arch Bridges: The arched shape of these bridges relies on compression members to transfer the loads to the supports and maintain the structural integrity of the arch.
- Cable-Stayed Bridges: The towers or pylons in cable-stayed bridges are designed as compression members to support the weight of the deck and resist lateral loads.
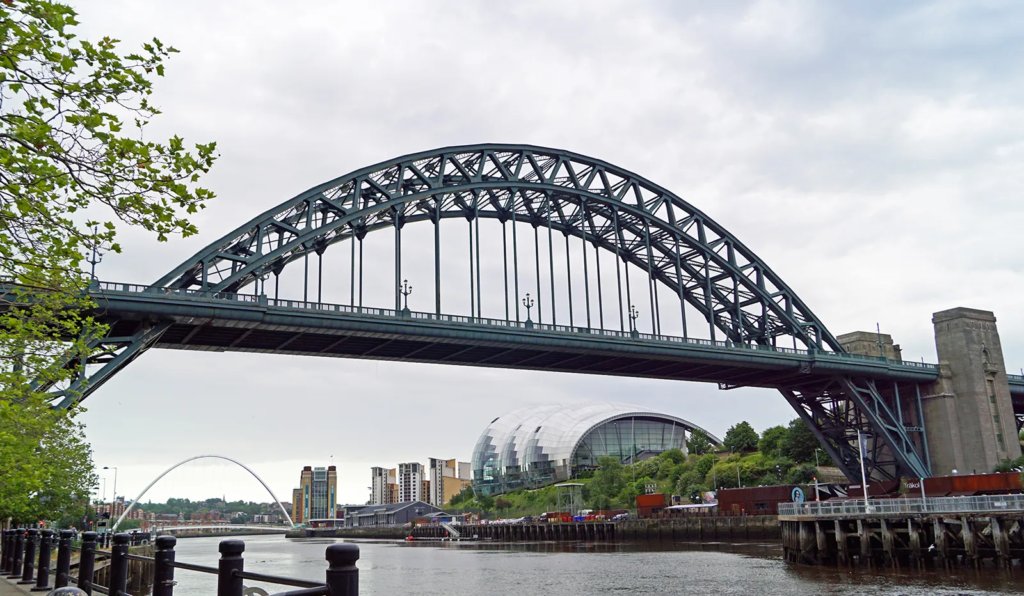
Industrial Structures
Examples and Design Considerations:
Compression members are extensively used in industrial structures, such as factories, warehouses, and power plants, where heavy loads and specialized requirements must be addressed. Examples include:
- Transmission Line Towers: These structures rely on compression members, such as lattice towers or tubular poles, to support the weight of the conductors and withstand wind and environmental loads.
- Storage Racks and Shelving Systems: Vertical columns and braces act as compression members, supporting the weight of stored goods and ensuring the stability of the racking system.
- Offshore Platforms: Compression members, such as jacket structures or piles, are crucial for supporting the deck and resisting environmental loads in offshore oil and gas platforms.
These case studies and real-world applications highlight the versatility and importance of compression members in various steel structures, as well as the need for careful design, analysis, and construction practices to ensure their performance and safety.
Innovative Technologies and Trends
The field of compression members in steel structures is continuously evolving, driven by advancements in materials, design tools, and construction techniques. These innovative technologies and trends aim to improve the performance, efficiency, and sustainability of steel structures.
Advanced Materials
High-Strength Steel:
The development of high-strength steel grades has revolutionized the design and construction of compression members. These materials offer higher yield and tensile strengths, allowing for reduced cross-sectional dimensions and weight optimization without compromising structural integrity. Examples include ASTM A913, A1085, and A1035 steels, which have yield strengths ranging from 65 ksi to 100 ksi.
Composite Materials:
The use of composite materials, such as fiber-reinforced polymers (FRP) or concrete-filled steel tubes, has gained traction in the construction industry. These materials offer enhanced strength-to-weight ratios, improved corrosion resistance, and tailored mechanical properties. Composite compression members can provide superior performance and durability compared to traditional steel members.
Modern Design Tools
Finite Element Analysis (FEA):
Finite Element Analysis (FEA) is a powerful computational tool that enables engineers to accurately model and analyze the behavior of compression members under various loading conditions. FEA simulations can account for complex geometries, material properties, and boundary conditions, providing insights into stress distributions, buckling behavior, and potential failure modes.
Building Information Modeling (BIM):
Building Information Modeling (BIM) is a digital representation of a construction project that integrates various aspects, including structural design, material specifications, and construction planning. BIM allows for efficient collaboration, clash detection, and optimization of compression member designs, reducing errors and improving overall project coordination.
Automation and Prefabrication:
Advancements in automation and prefabrication techniques have streamlined the manufacturing process of compression members. Automated welding, cutting, and assembly processes ensure consistent quality and reduce the potential for human error. Prefabricated components can be efficiently transported to construction sites, reducing on-site labor and construction time.
These innovative technologies and trends contribute to the ongoing development of compression members in steel structures, enabling more efficient, cost-effective, and sustainable designs while maintaining or enhancing structural performance and safety.
Compression members are critical components in steel structures, playing a vital role in transferring loads, providing stability, and ensuring the overall structural integrity. This comprehensive article has explored various aspects of compression members, from their types and materials to design principles, buckling behavior, and analysis codes.
Summary of Key Points:
- Compression members are classified into different types, including short columns, long columns, struts, braces, and built-up columns, each with specific characteristics and applications.
- Material selection for compression members is crucial, with structural steel grades like ASTM A36 and A992 being commonly used, considering properties such as yield strength, ultimate strength, and ductility.
- Design principles for compression members involve calculating load-carrying capacity, effective length, and slenderness ratio, while accounting for factors like axial load, buckling load, and end conditions.
- Buckling is a critical failure mode for compression members, with Euler buckling and inelastic buckling being the two main types. Factors like slenderness ratio, material properties, and end conditions influence buckling behavior.
- Bracing, cross-section modifications, and adherence to design codes and standards are essential for preventing buckling and ensuring the structural integrity of compression members.
- Common problems and solutions, such as local and global buckling, as well as connection failures, must be addressed through proper identification and strengthening techniques.
- Case studies and real-world applications, including high-rise buildings, bridges, and industrial structures, highlight the versatility and importance of compression members in steel structures.
- Innovative technologies and trends, such as advanced materials, finite element analysis, and building information modeling, are shaping the future of compression member design and construction.
Future of Compression Members in Steel Structures:
As the demand for taller, more efficient, and sustainable structures continues to grow, the role of compression members in steel structures will become increasingly important. Research and development in areas such as high-performance materials, advanced computational tools, and optimized design methodologies will drive the evolution of compression member design and construction.
Additionally, the integration of emerging technologies, such as additive manufacturing (3D printing) and robotics, may enable the fabrication of compression members with complex geometries and optimized load paths, leading to more efficient and lightweight designs.
Furthermore, the emphasis on sustainability and environmental consciousness will likely influence the selection and use of materials for compression members. The incorporation of recycled or reused materials, as well as the development of low-carbon steel production processes, could contribute to reducing the environmental impact of steel structures.
As our understanding of the behavior of compression members under various loading conditions continues to improve, design codes and standards will evolve to reflect the latest research findings and best practices. This will ensure that compression members in steel structures continue to meet the highest standards of safety, reliability, and performance.
Overall, the future of compression members in steel structures holds promise for innovative solutions that combine structural efficiency, sustainability, and technological advancements, enabling the construction of more resilient, cost-effective, and environmentally responsible structures.
Frequently Asked Questions (FAQs)
What is the difference between short and long columns?
The primary difference between short and long columns lies in their slenderness ratio, which is the ratio of the effective length to the least radius of gyration.
Short columns, also known as stocky columns, have a relatively low slenderness ratio. They are capable of resisting compressive forces primarily through direct compression, with minimal susceptibility to buckling effects. Short columns are typically used in applications where the primary load is compressive, such as lower levels of multi-story buildings or support columns for machinery.
On the other hand, long columns, or slender columns, have a higher slenderness ratio compared to short columns. Due to their increased slenderness, long columns are more susceptible to lateral deformations and buckling under compressive loads. Their design considerations must account for the potential for buckling failure modes. Long columns are often employed in structures where significant vertical loads need to be transferred over considerable heights, such as upper levels of high-rise buildings, transmission line towers, or offshore platforms.
How do you calculate the buckling load for a steel column?
The buckling load for a steel column can be calculated using various methods, depending on the column’s slenderness ratio and the applicable design code or standard. Here are two commonly used approaches:
- Euler Buckling Load (for slender columns):
The Euler buckling load is a theoretical value based on the principles of elastic stability and is applicable to slender columns with a high slenderness ratio. It is calculated using the following equation:
P_cr = (π^2 × E × I) / (K × L)^2
Where:
P_cr = Euler buckling load
E = Modulus of elasticity of the material
I = Moment of inertia of the cross-section
K = Effective length factor (depends on end conditions)
L = Effective length of the column
- Inelastic Buckling Load (for stocky columns):
For stocky columns with a lower slenderness ratio, inelastic buckling behavior becomes more prevalent, and the design codes provide specific equations or interaction formulas to determine the buckling load. The general approach involves calculating the critical stress based on the slenderness ratio and the yield strength of the material, and then multiplying it by the cross-sectional area to obtain the buckling load.
It’s important to note that the specific equations and design methodologies may vary depending on the applicable design code or standard, such as AISC, Eurocode, or other national or regional codes.
What are the common materials used in compression members?
The common materials used for compression members in steel structures are structural steel grades specified by various standards and codes. Some of the most widely used materials include:
- ASTM A36: A carbon steel grade with a minimum yield strength of 36 ksi (248 MPa), suitable for various compression members and general structural applications.
- ASTM A992: A high-strength, low-alloy steel grade with a minimum yield strength of 50 ksi (345 MPa), commonly used in compression members for high-rise buildings and structures subjected to heavy loads.
- ASTM A572: A high-strength, low-alloy steel grade available in various grades with yield strengths ranging from 42 ksi to 65 ksi (290 MPa to 450 MPa), used for compression members in various applications.
- ASTM A913: A high-strength, low-alloy steel grade with a minimum yield strength of 65 ksi (450 MPa), suitable for compression members in structures requiring higher strength and weight optimization.
- ASTM A500: A cold-formed, hollow structural steel tubing commonly used in built-up compression members and truss systems.
- Weathering Steel: Steel grades like ASTM A588 and A847, which develop a protective oxide layer and provide enhanced corrosion resistance, can be used in compression members for outdoor applications.
In addition to these structural steel grades, other materials such as concrete-filled steel tubes, fiber-reinforced polymers (FRP), or advanced high-strength steels may also be employed in compression members, depending on the specific project requirements and design considerations.
The selection of materials for compression members involves considering factors such as strength requirements, cost-effectiveness, durability, and compatibility with other structural components, while adhering to the applicable design codes and standards.
[…] article delves into the realm of steel structures, with a specific emphasis on their foundations. We will explore the advantages of steel as a […]