Winds and seismic loads are two of the most significant forces that structures must withstand during their lifespan. These natural phenomena have the potential to cause substantial damage or even collapse if not properly accounted for in the design and construction phases. As such, understanding and mitigating the effects of wind and seismic loads is crucial for ensuring the safety and longevity of buildings, bridges, and other structures.
Definition of winds and seismic loads
Wind loads refer to the lateral forces exerted on a structure due to the movement of air particles. These loads can vary in intensity and direction, depending on factors such as wind speed, terrain characteristics, and the shape and orientation of the structure itself. On the other hand, seismic loads are the forces generated by ground motions during an earthquake. These loads can cause structures to experience complex movements, including lateral, vertical, and torsional vibrations.
Importance in structural engineering
The consideration of wind and seismic loads is a fundamental aspect of structural engineering. Structures must be designed to resist these loads to prevent damage, collapse, and potential loss of life. Failure to account for these forces can have catastrophic consequences, as evidenced by numerous historical incidents where buildings and infrastructure were severely damaged or destroyed by high winds or earthquakes.
Overview of how they affect buildings and structures
Wind loads can exert significant lateral forces on a structure, causing it to sway or even overturn if not properly designed. These loads can also create uplift forces on roofs and overhanging elements, potentially leading to their detachment or failure. Seismic loads, on the other hand, can cause complex structural deformations and stress concentrations, leading to cracking, yielding, or even complete collapse of the structure. Additionally, seismic loads can amplify the effects of wind loads, creating a compound loading scenario that must be carefully considered in the design process.
Understanding Wind Loads
Factors influencing wind loads (velocity, direction, terrain, etc.)
Several factors contribute to the magnitude and distribution of wind loads on a structure. Wind velocity is a primary determinant, with higher wind speeds generally resulting in greater loads. The direction of the wind relative to the structure’s orientation also plays a crucial role, as certain orientations may be more susceptible to wind effects. Terrain characteristics, such as surface roughness and obstacles, can influence wind flow patterns and turbulence, affecting the loads experienced by the structure. Additionally, the structure’s shape, size, and aerodynamic properties can significantly impact how wind loads are distributed across its surfaces.

Wind load calculation methods (e.g., ASCE 7, Eurocode)
To accurately predict and design for wind loads, various calculation methods and standards have been developed. The American Society of Civil Engineers (ASCE) 7 standard is widely used in the United States and provides comprehensive guidelines for determining wind loads based on factors such as wind speed, exposure category, and building risk category. The Eurocode, on the other hand, is a set of standards used in Europe and other regions, which includes provisions for calculating wind loads based on wind speed, terrain characteristics, and structure height, among other factors.
Importance of wind load considerations in structural design
Proper consideration of wind loads is essential for ensuring the stability and safety of structures. Failure to account for these loads can lead to catastrophic consequences, such as structural damage, collapse, and potential loss of life. Wind loads can be particularly critical for tall buildings, bridges, and other structures with large surface areas exposed to wind effects. Neglecting wind load considerations can result in inadequate structural design, compromising the integrity and longevity of the structure.
Examples of structures affected by wind loads
Various types of structures can be significantly impacted by wind loads, including:
- High-rise buildings: Tall buildings are particularly vulnerable to wind loads due to their height and large surface area exposed to wind forces.
- Bridges: Long-span bridges, especially those with slender designs, can experience significant wind-induced vibrations and lateral loads.
- Stadiums and arenas: Large, open structures with expansive roofs or canopies are susceptible to uplift forces and lateral loads caused by wind.
- Industrial facilities: Structures such as cooling towers, chimneys, and storage tanks can be affected by wind loads due to their height and unique shapes.
Understanding Seismic Loads
Introduction to seismic activity and earthquakes
Seismic activity refers to the vibrations and ground motions caused by the release of energy within the Earth’s crust. Earthquakes are the most common and significant manifestation of seismic activity, resulting from the sudden movement of tectonic plates or ruptures along fault lines. These events can generate powerful seismic waves that propagate through the ground, causing structures to experience severe shaking and deformation.
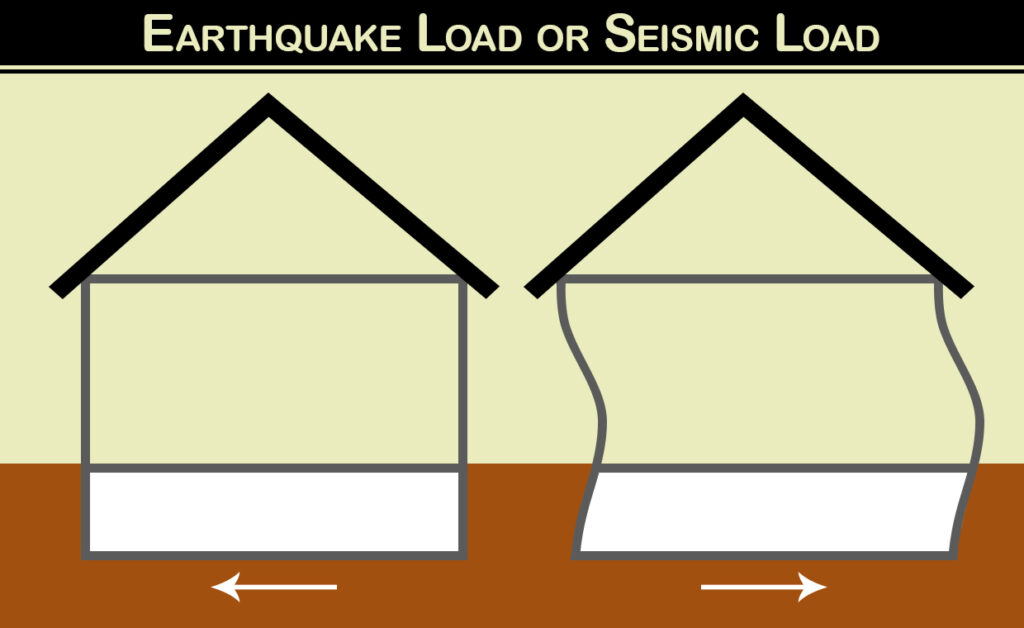
Factors influencing seismic loads (e.g., magnitude, soil type, building materials)
The magnitude of seismic loads experienced by a structure is influenced by several factors. The magnitude of the earthquake itself, measured on scales such as the Richter or Moment Magnitude scales, is a primary determinant of the seismic energy released and the resulting ground motions. Additionally, the soil type and local site conditions can amplify or attenuate seismic waves, affecting the intensity of shaking experienced by the structure. The building materials used in construction, as well as the structural system and configuration, also play a role in how seismic loads are transferred and distributed throughout the structure.
Seismic load calculation methods (e.g., Uniform Building Code, International Building Code)
To ensure structures can withstand seismic loads, various building codes and standards provide methodologies for calculating and designing for these forces. The Uniform Building Code (UBC) and the International Building Code (IBC) are two widely used codes in the United States that provide seismic design provisions based on factors such as seismic hazard maps, site soil conditions, and structural system specifications. These codes aim to ensure that structures can resist seismic loads while maintaining an acceptable level of performance and safety.
Importance of seismic load considerations in structural design
Proper consideration of seismic loads is crucial for ensuring the safety and resilience of structures in earthquake-prone regions. Failure to account for these loads can lead to catastrophic consequences, including structural damage, collapse, and potential loss of life. Seismic load considerations are particularly important for critical infrastructure, such as hospitals, fire stations, and bridges, which must remain operational during and after an earthquake event.
Examples of structures affected by seismic loads
Various types of structures can be significantly impacted by seismic loads, including:
- Buildings: Residential, commercial, and industrial buildings can experience severe shaking and deformation during earthquakes, leading to potential structural damage or collapse.
- Bridges: Bridges are particularly vulnerable to seismic loads due to their spanning nature and the potential for differential ground motions at their supports.
- Dams and levees: These structures can be subjected to significant seismic loads, which can lead to failures and potentially catastrophic flooding.
- Nuclear power plants: The safety and integrity of nuclear facilities must be maintained during seismic events to prevent the release of radioactive materials.
READ MORE HERE Designing For Seismic Resilience Reinforced Concrete Structures
Comparison of Wind and Seismic Loads
Differences in behavior and effects on structures
While both wind and seismic loads can exert significant forces on structures, their behavior and effects differ in several ways. Wind loads typically apply lateral forces that can cause swaying or overturning of structures, while seismic loads induce complex motions, including lateral, vertical, and torsional vibrations. Additionally, wind loads are generally more sustained and consistent in nature, while seismic loads are transient and characterized by high-frequency ground motions.
Similarities in calculation methods and design considerations
Despite their differences, wind and seismic load calculations share some similarities in their approaches. Both rely on hazard maps or models that consider factors such as wind speed or seismic activity, as well as site-specific characteristics like terrain or soil conditions. Furthermore, design considerations for both types of loads often involve strategies to increase structural stiffness, strength, and ductility to resist the imposed forces.
How wind and seismic loads may interact in certain situations
In certain situations, wind and seismic loads can interact and compound their effects on structures. For example, during an earthquake, structures may already be experiencing lateral deformations and stresses due to seismic loads, making them more vulnerable to the additional lateral forces imposed by wind loads. Conversely, high wind loads can potentially amplify the effects of seismic loads by inducing additional sway or torsional movements in structures. These compounded loading scenarios must be carefully considered in the design process to ensure structural integrity and safety.
Design Considerations for Wind Loads
Structural elements vulnerable to wind loads (e.g., roof, walls, windows)
Several structural elements are particularly vulnerable to wind loads and require special consideration in the design process:
- Roof systems: Roofs are susceptible to uplift forces caused by wind, which can lead to detachment or failure if not properly secured and designed to resist these loads.
- Exterior walls: Wind loads can exert significant lateral pressures on exterior walls, potentially causing cracking, deformation, or even collapse if inadequately reinforced or braced.
- Windows and glazing systems: Large window surfaces can experience significant wind pressures, leading to potential glass breakage or frame failure if not designed to withstand the anticipated wind loads.
- Cladding and exterior finishes: Wind loads can cause detachment or damage to cladding materials, such as metal panels or masonry veneers, if not properly secured and anchored.
Strategies for mitigating wind effects (e.g., aerodynamic shapes, windbreaks)
Several strategies can be employed to mitigate the effects of wind loads on structures:
- Aerodynamic shaping: Incorporating aerodynamic shapes and features, such as rounded corners or tapered forms, can help reduce wind pressures and minimize turbulence around the structure.
- Windbreaks and sheltering: Strategically placing windbreaks or surrounding structures can help shield a building from direct wind exposure, reducing the overall wind loads experienced.
- Structural bracing and reinforcement: Incorporating adequate bracing, shear walls, and reinforcement can increase a structure’s lateral stiffness and ability to resist wind-induced deformations and stresses.
- Dynamic response modification: Implementing mechanisms such as tuned mass dampers or base isolation systems can help dissipate wind-induced vibrations and reduce the overall dynamic response of the structure.
Case studies of buildings designed to withstand high wind loads
Several notable buildings have been designed specifically to withstand extreme wind loads:
- Burj Khalifa, Dubai: The world’s tallest building (828 meters) was designed to resist wind loads through its unique Y-shaped reinforced concrete core and aerodynamic shape, which helps minimize vortex shedding and wind pressure.
- Shanghai Tower, Shanghai: This 632-meter-tall skyscraper features a unique twisted form and a reinforced concrete core that provides lateral stiffness against wind loads. The building’s aerodynamic shape also helps reduce wind pressures.
- Taipei 101, Taipei: At 508 meters tall, this iconic tower was designed with a tuned mass damper system to mitigate wind-induced vibrations. The building’s reinforced concrete core and outrigger truss system also contribute to its wind resistance.
- Petronas Towers, Kuala Lumpur: These iconic twin towers, each standing at 452 meters, were designed with a unique structural system that incorporates a central concrete core, outrigger trusses, and belt trusses, providing robust resistance against wind loads.
Design Considerations for Seismic Loads
Seismic-resistant design principles (e.g., base isolation, damping systems)
Several design principles and strategies are employed to enhance a structure’s resistance to seismic loads:
- Base isolation: This technique involves decoupling the structure from the ground motion by introducing a flexible interface, such as rubber bearings or sliding bearings, between the foundation and the superstructure. This helps reduce the seismic forces transmitted to the building.
- Damping systems: Various damping systems, such as viscous dampers, friction dampers, or tuned mass dampers, can be incorporated into a structure to dissipate seismic energy and reduce the amplitude of vibrations.
- Ductile design: Structures are designed with ductile materials and detailing techniques that allow for controlled deformation and energy dissipation during seismic events, preventing brittle failures.
- Shear walls and bracing systems: Incorporating shear walls, braced frames, or other lateral load-resisting systems can increase a structure’s lateral stiffness and ability to resist seismic forces.
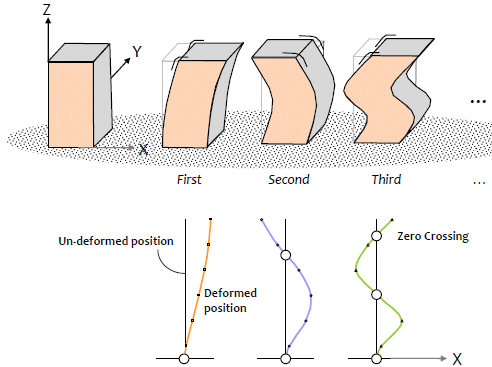
Importance of building codes and regulations in seismic-prone regions
Building codes and regulations play a crucial role in ensuring seismic resilience in earthquake-prone regions. Codes such as the International Building Code (IBC) and the Uniform Building Code (UBC) provide specific seismic design requirements based on the expected seismic hazard levels and the occupancy and importance of the structure. These codes mandate minimum design standards, material specifications, and detailing requirements to ensure adequate seismic performance.
Compliance with these codes is essential for protecting public safety and minimizing the risk of structural failures during seismic events. Additionally, many regions have their own localized building codes and regulations that account for specific seismic hazards and construction practices.
Retrofitting existing structures for improved seismic resilience
While new construction can incorporate seismic-resistant design principles from the outset, existing structures may require retrofitting to enhance their seismic resilience. Retrofitting techniques may include:
- Strengthening and bracing: Adding shear walls, braced frames, or other lateral load-resisting systems can improve a structure’s ability to resist seismic forces.
- Base isolation or energy dissipation systems: Implementing base isolation or damping systems can help decouple the structure from ground motions and dissipate seismic energy.
- Material upgrades: Replacing or reinforcing structural elements with higher-strength or more ductile materials can improve seismic performance.
- Foundation upgrades: Strengthening or modifying the foundation system to better transfer seismic loads to the ground can enhance overall structural resilience.
Retrofitting existing structures is often more challenging and costly than incorporating seismic-resistant design principles during initial construction. However, it remains a crucial step in ensuring the safety and longevity of existing buildings and infrastructure in seismic-prone regions.
Case studies of buildings that have successfully withstood earthquakes
Several notable buildings have demonstrated impressive seismic resilience during major earthquake events:
- Sendai Mediatheque, Sendai, Japan: This innovative structure, designed by architect Toyo Ito, survived the 2011 Tohoku earthquake (magnitude 9.0) with minimal damage due to its advanced seismic-resistant design, including base isolation and a unique structural system.
- U.S. Bank Tower, Los Angeles, USA: This 73-story skyscraper, formerly known as the Library Tower, withstood the 1994 Northridge earthquake (magnitude 6.7) without significant structural damage, thanks to its reinforced concrete core, outrigger trusses, and base isolation system.
- Transamerica Pyramid, San Francisco, USA: The iconic Transamerica Pyramid, completed in 1972, successfully weathered the 1989 Loma Prieta earthquake (magnitude 6.9) due to its reinforced concrete core and innovative structural design that incorporated seismic considerations.
- Mexico City Hospital, Mexico City, Mexico: This hospital complex, designed with base isolation and energy dissipation systems, remained operational and sustained minimal damage during the 2017 Puebla earthquake (magnitude 7.1), demonstrating the effectiveness of advanced seismic-resistant design principles.
Integrated Design Approach for Wind and Seismic Loads
Importance of considering both loads simultaneously in structural design
While wind and seismic loads are often considered separately in structural design, it is crucial to adopt an integrated approach that accounts for the potential interaction and compounded effects of these loads. Failure to consider both loads simultaneously can lead to inadequate design and increased risk of structural failure.
Structures located in regions prone to both high winds and seismic activity must be designed to withstand the combined effects of these loads. For example, during an earthquake, a structure may already be experiencing lateral deformations and stresses, making it more vulnerable to the additional lateral forces imposed by wind loads. Conversely, high wind loads can potentially amplify the effects of seismic loads by inducing additional sway or torsional movements in structures.
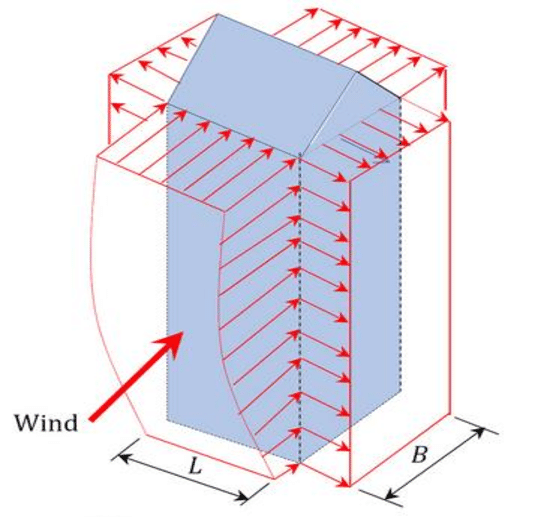
By considering wind and seismic loads simultaneously, designers can account for the potential compounding effects and ensure that the structure is adequately designed to resist the combined loading scenarios.
Strategies for integrating wind and seismic load considerations
Several strategies can be employed to integrate wind and seismic load considerations in structural design:
- Performance-based design: Adopting a performance-based design approach that considers multiple hazards, including wind and seismic loads, can help ensure that the structure meets specific performance objectives under various loading scenarios.
- Coupled analysis: Performing coupled analyses that consider the simultaneous effects of wind and seismic loads can provide a more accurate representation of the structural response and facilitate the design of appropriate mitigation measures.
- Redundancy and robustness: Incorporating redundant load paths and robust structural systems can enhance a structure’s ability to resist the combined effects of wind and seismic loads, even if localized damage occurs.
- Advanced computational tools: Utilizing advanced computational tools, such as finite element analysis and computational fluid dynamics, can aid in accurately modeling and simulating the complex interactions between wind and seismic loads on structures.
- Multidisciplinary collaboration: Fostering collaboration between structural engineers, wind engineers, seismologists, and other relevant disciplines can lead to a more comprehensive understanding of the combined effects and facilitate the development of integrated design solutions.
Examples of structures designed with a holistic approach to withstand both types of loads
While many structures are designed primarily to resist either wind or seismic loads, some notable examples have employed a holistic approach to address both types of loads:
- Burj Khalifa, Dubai: In addition to its wind-resistant design features, such as its aerodynamic shape and reinforced concrete core, the Burj Khalifa also incorporates seismic-resistant elements, including base isolation and reinforced concrete shear walls, to withstand potential earthquakes in the region.
- Tokyo Skytree, Tokyo: This 634-meter-tall broadcasting tower was designed to resist both high winds and seismic loads. Its triangular cross-section and reinforced concrete core provide lateral stiffness against wind loads, while its foundation and structural systems incorporate seismic-resistant design principles.
- Singapore Sports Hub: The Sports Hub complex, which includes a retractable roof stadium and other facilities, was designed to withstand both typhoon-level wind loads and seismic loads. The structures feature robust structural systems, energy dissipation devices, and base isolation to mitigate the effects of both types of loads.
- San Francisco-Oakland Bay Bridge, USA: The new East Span of this iconic bridge incorporates numerous features to resist both wind and seismic loads, including aerodynamic deck sections, seismic energy dissipation devices, and a self-anchored suspension span designed to withstand a maximum credible earthquake.
By employing an integrated design approach that considers both wind and seismic loads, these structures demonstrate the ability to withstand multiple natural hazards, ensuring greater resilience and longevity.
Wind and seismic loads are two of the most significant forces that structures must withstand, with the potential to cause substantial damage or even collapse if not properly accounted for. Understanding the factors influencing these loads, their calculation methods, and their effects on structures is crucial for effective structural design.
Wind loads are lateral forces exerted by the movement of air particles, influenced by factors such as wind speed, terrain characteristics, and the structure’s shape and orientation. Seismic loads, on the other hand, are generated by ground motions during earthquakes, causing complex lateral, vertical, and torsional vibrations.
While wind and seismic loads have distinct characteristics, they can interact and compound their effects in certain situations, necessitating an integrated approach to design considerations and mitigation strategies.
Importance of proper design and engineering in ensuring structural safety
Proper design and engineering practices are essential for ensuring the safety and longevity of structures in the face of wind and seismic loads. Failure to account for these loads adequately can lead to catastrophic consequences, including structural damage, collapse, and potential loss of life.
Adherence to building codes and regulations, particularly in wind and seismic-prone regions, is crucial for meeting minimum design standards and protecting public safety. Additionally, incorporating advanced design principles, such as base isolation, damping systems, and aerodynamic shaping, can significantly enhance a structure’s resilience against these natural hazards.
Future trends and advancements in wind and seismic load analysis and design
As our understanding of wind and seismic phenomena continues to evolve, and computational capabilities advance, several trends and advancements are expected in the analysis and design of structures subjected to these loads:
- Improved hazard modeling and mapping: Advances in remote sensing, data collection, and computational modeling will lead to more accurate and comprehensive hazard maps for wind and seismic loads, enabling more precise risk assessments and design considerations.
- Performance-based design methodologies: There is a growing emphasis on performance-based design approaches that consider multiple hazards and allow for quantitative evaluation of a structure’s performance under various loading scenarios, enabling more robust and resilient designs.
- Advanced computational tools and simulations: The increasing availability and power of computational tools, such as high-performance computing and machine learning, will enable more accurate and detailed simulations of wind and seismic load effects on structures, facilitating optimized design solutions.
- Innovative materials and systems: The development of new materials, such as high-performance concrete and advanced composites, as well as innovative structural systems and technologies, like adaptive damping systems and self-centering systems, will provide engineers with more tools to mitigate the effects of wind and seismic loads.
- Integrated monitoring and control systems: The integration of structural health monitoring systems and active control systems will enable real-time monitoring and adaptive response to wind and seismic loads, enhancing the resilience and safety of structures throughout their lifespan.
By embracing these trends and advancements, the structural engineering community can continue to push the boundaries of wind and seismic load analysis and design, creating safer, more resilient, and sustainable structures for the future.